|
 |
THE CERBRAL CORTEX
Phylogenetically, the human cerebral cortex is composed of a
relatively recent and extensive portion, the neocortex, and an
older relatively small region, the allocortex. The allocortex
comprises only about 10 percent of the total cortical area and
is limited to the olfactory cortex and the cingulate,
parahippocampal and dentate gyri. It is functionally subordinate
to the much larger neocortex, which comprises almost 90 percent
of the cortex and represents almost all of the highly convoluted
hemispheres seen in the exposed brain. The neocortex is composed
of six distinguishable layers (laminae) which vary in thickness
and density from one cortical region to another. The laminae are
distinguishable from each other by the cell types found in each
and by the type and direction of fibers which pass through them.
The laminae are numbered from I to VI. with I being at the
cortical surface and the others lying progressively deeper. The
six laminae are described in Table -1.
Tabe-1
Neocortical Laminae |
Lamina |
Layer |
Description |
I |
Molecular |
Primarily
composed of the sparsely scattered horizontal cells of
Cajal and the horizontal fibers of pyramidal cells,
stellate cells, and cells of Martinotti. |
II |
External granular |
Composed of
densely packed stellate and small pyramidal cells. This
area is traversed by
vertical
fibers from both ascending axons and apical dendrites of
large pyramidal cells in lamina V. The ascending axons
often synapse with the apical dendrites in this layer. |
III |
External pyramidal |
Medium-sized
pyramidal cells are located here. Stellate and basket
cells are also present. |
IV |
Internal granular |
This layer
is characterized by stellate cells and small pyramidal
cells. The external band of Baillarger, a
concentrated band of horizontal fibers, also runs
through this layer. |
V |
Internal pyramidal |
This is a
dense layer composed of large pyramidal cells. It is
also characterized by many ascending and descending
fibers. A horizontal band of concentrated fibers, the
internal band of Baillarger, traverses this layer. |
VI
|
Multiform |
Most of the
cells in this layer are small and represent a variety of
morphological types. |
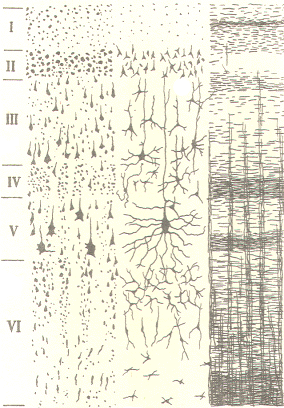 |
Cellular structure of the cerebral
cortex |
Physiologists often subdivide the cerebral cortex into regions
based on the functional characteristics of cortical layers in
that region. Typically included are the sensory cortex (koniocortex),
association cortex (homotypical cortex), and the motor cortex
(heterotypical cortex). The sensory cortex includes the
principal sensory receiving areas. while the association cortex
covers major portions of the brain including the frontal,
parietal, and temporal lobe. The motor cortex includes the
principal motor areas. The relative thickness of cortical
laminae IV and V is the noticeably variable feature between the
three regions. The internal granular layer (IV) is the main
receiving area for the sensory projection fibers from the
thalamus. Consequently lamina IV is thickest in the sensory
cortex. The internal pyramidal layer (V) is characterized by
large pyramidal cells whose descending axons represent the motor
fibers of the corticospinal system. Not surprisingly lamina V
is largest in the motor regions of the cortex. Both laminae
appear to be equally important in the association cortex as it
receives some sensory input and gives rise to some motor output.
It is also important to note that while the sensory cortex is
primarily concerned with sensory input, it does give rise to a
small motor component. Likewise the motor cortex receives a
small degree of sensory input.
The
circuitry of the cerebral cortex has been much more difficult to
evaluate than that of the cerebellar cortex. Because of the
dense nature of neuronal elements, the extensive nature of
dendritic processes called neutropil, and lack of repetitious
patterns of neuronal contacts, meaningful evaluation of
cortical neuronal circuitry has been difficult and not very
fruitful. Recall that the neuronal makeup of the cerebellar
cortex is everywhere identical and shows very symmetrical and
repeated patterns. This, coupled with low neuronal density in
the cerebellar cortex, has made experimentation and evaluation
of cerebellar circuitry much easier than is true for the
cerebral cortex. Another factor related to the difficulty of
examining cerebral cortical circuitry is that fibers afferent to
the cortex do not show the same consistency in their
terminations as seen in the cerebellar cortex. Recall the
climbing fiber-Purkinje cell and mossy fiber-granular cell
synapses observed in the latter. Nevertheless, the efferent
output from the cerebral cortex is primarily through axons of
pyramidal cells in laminae II to V with the largest cells in
lamina V. Cortical afferents project to all six laminae, with
lamina IV of the sensory cortex receiving the largest number of
collateral synapses.
 |
Brodmann's Areas
|
Brodmann, an early twentieth-century German neurologist,
described the sixlayered cortex just discussed. Using Nissl
stain, which clearly shows cell bodies but not neurites, he
identified six distinct layers. Later work utilizing Golgi and
Weigert stains brought out additional detail not previously
seen. Brodmann mapped the cerebral cortex into many areas based
on variations in the six layers. Many attempts have been made by
physiologists to ascribe specific functional importance to these
areas. In some cases this has been possible (e.g.,
Brodmann's area 17 and the primary visual cortex), but in many
cases no distinct relationship exists. More often than not,
specific functional regions seem to overlap several areas.
Nevertheless, Brodmann's areas are quite useful as landmark
indicators because of their worldwide recognition. The cortical
areas of Brodmann are illustrated in the lateral and median
sagittal views of Fig-1.
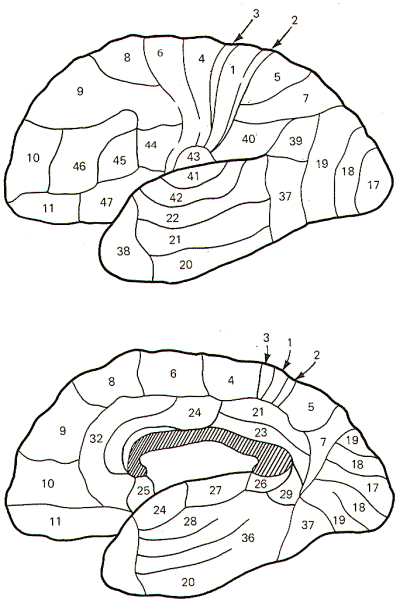 |
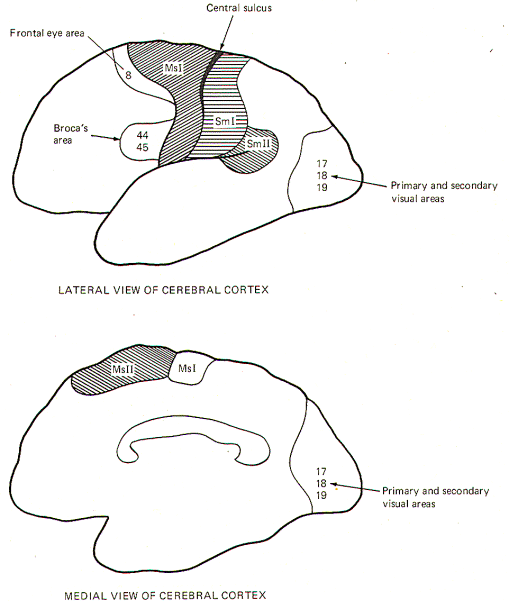 |
 |
Electrophysiological Studies of the Cortex |
Most of what we know concerning the functional role of the
cortex is based on electrophysiological studies. Carefully
probing the cortex with a stimulating electrode and observing
the muscular responses produced has been the most useful
technique to uncover those cortical areas capable of initiating
movement. Similarly, stimulating peripheral receptors and
recording their "evoked responses" with cortical recording
electrodes has enabled researchers to determine those cortical
areas which receive sensory input. Not surprisingly there is
considerable overlap of the "motor" and "sensory" cortical
areas. Those areas adjudged to be motor because they produce
muscular movement when electrically stimulated are also capable
of generating evoked responses when peripheral receptors are
stimulated. Likewise, the "sensory" areas show a small motor
component as well. Nevertheless, one function seems to
predominate, and this has led to the establishment of a commonly
used classification scheme
which we will examine now.
 |
Motor Areas of
the Cortex
|
The motor areas of the cerebral cortex include the primary motor
area (MsI), secondary motor area (MsII), frontal eye area, and
Broca's motor speech area. While there may be others, these are
certainly the most demonstrable (Fig-2).
The Primary Motor Area (MsI) This area is located immediately
anterior to the central sulcus. Electrical stimulation of the
cortex in this region produces movements in the appropriate part
of the body on the contralateral side.
|
Fig-1 |
Fig-2 |
|
It is important to note
that movements initiated in this way are not single
uncoordinated contractions of given muscles, but rather
movements accompanied by contraction of agonists and relaxation
of antagonists. Nevertheless, these movements are very simple,
and are similar to those which might be produced by an infant.
Obviously more advanced movements must require the
incorporation of additional systems.
The primary motor area (equivalent to Brodmann's area 4 and an
adjacent strip of area 6) extends over the superior medial
border of the hemisphere onto the medial surface. The body is
represented as a homunculus with the head and face regions
located near the lateral fissure and the leg and foot areas
extending onto the medial surface. The back extends anteriorly
over area 4 onto the adjacent strip of area 6. The fingers and
toes extend over the cortical surface in the central sulcus.
Area MsI also has a small sensory component which receives input
from a number of sources. The lemniscal system to the VPL
nucleus of the thalamus ultimately projects from this nucleus to
area 4 of MsI. The cerebellum projects to the VL nucleus of the
thalamus, which in turn projects to areas 4 and 6 of MsI.
Finally, the globus pallidus sends fibers to both the V A and VL
nuclei of the thalamus which then project to area 6 of MsI. Much
of the input to MsI is proprioceptive, but sensory input from
other sources is also noted.
The Supplementary Motor Area (MsII) The extension of area 6 onto
the medial surface of the cortex represents the supplementary
motor area (MsII). The body is represented horizontally here
with the head forward, the back region lying adjacent to the
cingulate gyrus, and the fingers just reaching the upper surface
of the hemisphere. Electrical stimulation of this area produces
somewhat complex bilateral avoidance movements. They are not as
specifically distinct as those produced by MsI stimulation. The
VA and VL nuclei of the thalamus both project sensory input to
MsII. Both nuclei receive input from the globus pallidus, while
the cerebellum projects only to the VL nucleus.
The
Frontal
Eye Area: This region coincides with area 8. Electrical
stimulation here produces deviation of the eyes, head, and neck
to the opposite side.
Broca's Motor Speech Area: This area corresponds roughly to areas
44 and 45 of the frontal lobe. Most of our information
concerning its role comes from ablation studies and stimulation.
Curiously, the left hemisphere appears to be dominant as
ablation here usually abolishes sound production and often
produces a motor aphasia or speech paralysis in humans. The
effects typically aren't observed with ablation of the same area
in the right hemisphere. It is estimated that 90 percent of us
are left hemisphere dominant in this respect, regardless of
right- or left-handedness.
 |
Sensory Areas of the
Cortex
|
The Primary Somatic Sensorimotor Area (SmI) Areas 3, 1, and 2
produce the largest evoked responses when somatic sensory
receptors are stimulated. Smaller responses are recorded in the
primary motor area (MsI) of the precentral gyrus. Input from
touch, pressure, pain, temperature, and proprioceptors projects
to the VPL nucleus of the thalamus, which then projects heavily
to SmI, truly the principal receiving area for somatic
sensation.
The body homunculus represented in SmI is essentially a mirror
image of that found in the primary motor area (Msl) anterior to
the central sulcus. Studies with monkeys have indicated that
the topographic organization of SmI consists of a series of
overlapping bands at right angles to the central sulcus. Each of
these bands is the cortical area for the representation of a
single dermatome.
It is also now well established that electrical stimulation of
the postcentral gyrus (SmI) produces motor responses as well. It
was originally thought that such movements were the result of
current spread from the stimulating electrodes to the precentral gyrus. However, it is now quite clear that this is
not the case and that SmI is capable of producing motor
responses on its own, although requiring higher stimulation
intensities than MsI.
The Secondary Somatic Sensorimotor Area (SmII) This area,
located immediately posterior to the face region of SmI, is
characterized by a homunculus with the head represented
anteriorly, the leg muscles posteriorly, the back inferiorly,
and the hands and feet superiorly. Stimulation here causes gross
movements of postural adjustments which are diffuse and
widespread.
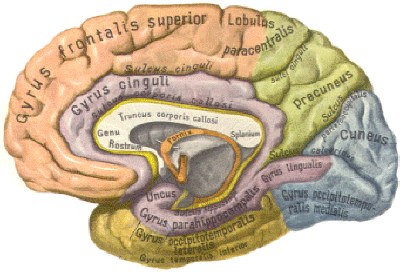 |
The Visual Cortex
A large area over the occipital pole of the
occipital lobe (areas 17, 18, and 19) represents the visual
cortex. Included are two functionally different areas: the
primary visual area and the visual association area. The
primary visual area (area 17) is the principal receiving
area for visual signals projected along the optic radiation
from the lateral geniculate body (LG). Recall that viewed
objects in the left visual field of both eyes project to the
right hemisphere while those in the right visual field project
to the left hemisphere. Further, objects viewed in the lower
quadrants of each visual field give rise to images in the cuneal
regions of area 17 while those in the upper quadrants project
images to the lingular regions.
Total ablation of area 17 renders a person blind. Nevertheless,
more than area 17 is required in order to comprehend the image
in the visual field which is projected on the visual cortex.
Areas 18 and 19 immediately surround the primary visual area and
comprise the visual association area. This area applies
cognition to the visual signal and helps to "make sense" out of
the projected image. |
|
|
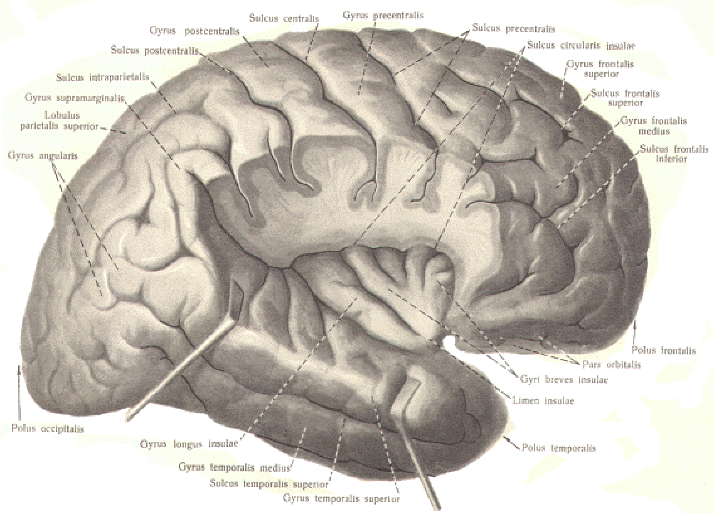 |
The Auditory Cortex This region includes the middle portion of
the superior temporal gyrus of the temporal lobe and a good
portion of the insular cortex. Like the visual cortex, it is
composed of two functionally different areas: the primary
auditory area and auditory association area. The primary
auditory
area
is a relatively small region in the middle of the superior
temporal convolution, extending over the superior surface to
part of the lateral and medial lip. This is roughly equivalent
to area 41. The medial geniculate body relays signals to this
area in response to input over the conscious auditory pathway.
When auditory impulses reach this area, the sound is heard but
not fully comprehended. Comprehension requires the participation
of the auditory association area. This area, covering the
insular cortex and a region surrounding the primary area on the
lateral surface of the temporal lobe (areas 42 and 22), has
extensive interconnections with area 41. |
|
|
The Gustatory Cortex This area is located near the most inferior
lateral extension of the primary sensorimotor area (Sml ) and
may include area 43. Taste signals relayed through the VPM
nucleus of the thalamus project to this area.
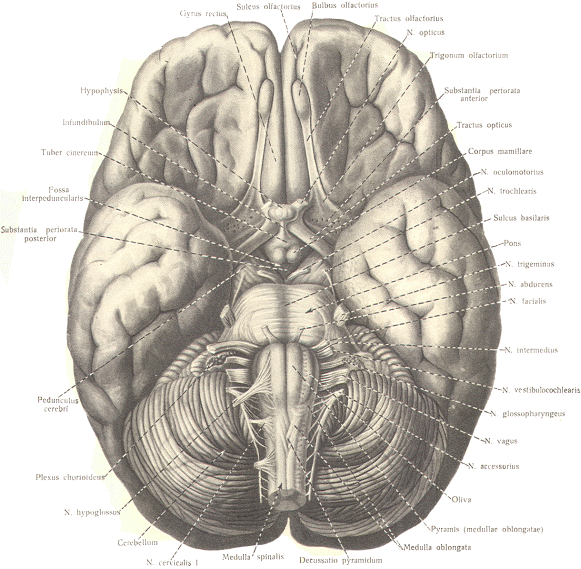 |
The Olfactory Cortex
You will recall that the olfactory tracts
divide into a medial and lateral stria as they approach the
anterior perforated substance. The lateral olfactory tract
terminates in the prepyriform cortex and parts of the amygdala
of the temporal lobe. These areas represent the primary
olfactory cortex. Fibers then project from here to area 28.
the secondary olfactory area, for sensory evaluation. The
medial olfactory tract projects to the anterior perforated
substance, the septum pellucidum, the subcallosal area. and even
the contralateral olfactory tract. Both the medial and lateral
olfactory tracts contribute to the visceral reflex pathways
causing the viscerosomatic and viscerovisceral responses. It is worth pointing out that unlike other
sensory systems, no relay through a thalamic nucleus occurs in
the olfactory system. |
|
|
 |
Cerebral
Dominance |
Certain behavioral patterns appear to be associated with one or
the other hemisphere. These include handedness, the performance
of speech, understanding the spoken and written word, and
spatial appreciation. Approximately 90 percent of adults are
right-handed (controlled by the left cerebral hemisphere) and
over 96 percent of adults have their speech centers (Broca's
motor speech area) located in the left hemisphere. This is
evidenced by the fact that almost all aphasic patients with
speech disorders have left-hemisphere lesions in Broca's area.
Additional studies with humans have clearly established that the
left hemisphere is best suited for written and oral language
expression as well as analytic calculation, while the right
hemisphere is particularly suited for appreciation of spatial
relationships and aesthetic and nonverbal expression. Thus, the
left hemisphere is often called the major or dominant
hemisphere, while the right is the minor hemisphere. It should
be noted that in those individuals who are left-handed, there is no similar shift in control from left to
right hemisphere of the other behavioral observations listed
above.
Split-Brain Studies The commissural fibers (corpus callosum and
anterior, posterior, and hippocampal commissures) serve to
connect the two hemispheres. We have learned a great deal about
the different functions of the two hemispheres from individuals
who have had a complete sectioning of the corpus callosum in
order to prevent the spread of epileptic seizures from one
hemisphere to the other. These "split-brain" individuals retain
normal behavioral patterns and can perform and learn as well as
normal people. However, cerebral dominance shows up in carefully
planned experiments.
Much of this work has been performed by R. W. Sperry. If an
unfamiliar object is placed in the left hand of blindfolded
split-brain individuals, they will be able to fully appreciate
its shape and touch by feeling it but will be unable to orally
describe it or accurately draw a picture of it with their right
hand. They cannot describe it orally because the right
hemisphere, which received the sensory input from the object,
is unable to communicate with the speech area of the dominant
left hemisphere. Similarly, they will be unable to accurately
draw it with the right hand because the important spatial
information received by the right hemisphere cannot be
transmitted to the dominant hemisphere. Thus, the apparent role
of the cerebral commissures is the bilateral hemispheric
integration of written and oral expression.
Visual input to the split-brain individual likewise demonstrates
cerebral dominance if carefully designed tests are performed. As
the reader will recall, objects viewed in the left visual fields
of both eyes are transmitted to the right occipital lobe. One
such test involves having the individual look straight ahead at
a table upon which are laid a variety of common objects such as
a paper clip, screwdriver, bottle, key, etc. If a card bearing
the printed name of one of these objects is flashed for 0.1 s in
the left visual field, the individual is quite successful when
asked to reach out and take the item named on the card with the
left hand. This is so because the right hemisphere received the
visual signal. and this same right hemisphere directs the
movement of the left hand to the appropriate item. The subject
could even crudely write the name of the retrieved item by
writing with the left hand, since the right hemisphere, which
received the signal, directs the activity of the left hand.
However, because of the failure of the right (minor) hemisphere
to communicate its information to the left (major) hemisphere
because of the severed commissure, the individual cannot
verbally say what name was seen on the card or what item was
retrieved by the left hand. The left "speaking" hemisphere has
not been informed of the actions of the right hemisphere.
Indeed, the individual verbally denies even seeing such a card.
Similarly, if asked to use the right hand to write the name of
the item retrieved with the left hand, the subject would be
unable to do so because the left "writing" hemisphere received
none of relevant information.
Identical results have now been observed in individuals who have
their brains essentially "chemically split" by the injection of
short-acting anesthetics into the left carotid artery, which
anesthetizes the left hemisphere.
 |
Evaluation of
Cortical Areas
by Lesion
Studies |
Much of our knowledge of the behavioral role of the various
cortical areas has been obtained by accidental or
disease-produced lesions in the human cerebral cortex. For
example, lesions in areas 18 and 19 don't produce blindness, as
visual signals still reach area 17 and objects can be clearly
seen, but they are neither recognized identified, nor
understood, This condition is known as visual agnosia,
meaning that the viewed object is "not known." Lesions limited
to area 17, however, produce outright blindness.
Lesions to Broca's motor speech area (44 and 45) in the major
hemisphere cause an expressive or motor aphasia.
The patient can't speak intelligibly. He or she knows what to
say but can't do it. There is no paralysis of the muscles
themselves, but the patient speaks very slowly often leaving out
nouns and verbs and has considerable difficulty with phrases.
The caudal aspect of the superior temporal gyrus, known as
Wernicke's area (area 22), is important for understanding
the spoken word. Lesions to this area, typically in the major
hemisphere, leave individuals able to hear normally, but spoken
words appear to be meaningless. Such people can speak but make
grammatical errors because of their failure to understand their
own spoken words. This region receives many fibers from other
association areas, including visual (18 and 19), auditory (41 and
42), and somesthetic (5 and 7). The condition, auditory
aphasia, is most severe if the lesion involves both
hemispheres, leaving these individuals unable to communicate
orally in any intelligible fashion.
The
angular gyrus
(area 39) is located at the caudal end of the lateral fissure
between the supramarginal gyrus and Wernicke's area. Like
Wernicke's area it has extensive connections with visual,
auditory, and somesthetic association areas. A lesion of area
39 in the major hemisphere leaves the individual unable to
comprehend written language. He or she can see words, but cannot
understand them. This inability to read (alexia) does not
prevent the individual from speaking normally but is usually
accompanied by the inability to write (agraphia).
Lesions to area 40, the supramarginal gyrus, inflicts a
person with tactile and proprioceptive losses. This individual
demonstrates astereognosis (the inability to identify
familiar objects by touch) and makes errors in judgment
concerning body position.
While we can learn much about the contribution made by
particular areas of the cerebral cortex from such lesion
studies, it is important to recognize that the areas involved
may simply be "links" in a chain as far as their affected
sensorimotor observations are concerned. Other cortical areas
may also be heavily involved. Further, eventually unraveling the
sequences of cortical area involvement in a given behavioral
pattern will still leave this greater mystery unsolved:
What is the pattern of neuronal sequencing and synaptic
integration?
 |
The Corticomotor
Reflex |
Current research supports a theory that the motor cortex is
composed of narrow, deep columns which represent the functional
units around which sensorimotor activity is organized. These
columns extend vertically through the entire six-layered
cortex. Each may be as narrow as a single millimeter. Part of
the basis for the columnar idea is based on observations that
the cutaneous receptive field of a given column of cortical
neurons lies in the path of movement produced by electrical
stimulation of this same column. Thus as the muscles of a limb
contract, objects encountered by the leading edge of the moving
limb may possibly stimulate cutaneous receptors which reflexly
reinforce the movement by projecting back to the same cortical
column. Therefore, cutaneous inputs may be constantly providing
feedback to guide limb movements via this corticomotor reflex.
It is possible that this feedback may also involve muscle and
joint receptors, causing the reflex to behave somewhat like a
tracking system by directing the limb to follow the path of tactile
and proprioceptive stimulation.
The corticomotor reflex may also behave much like the stretch
reflex in providing background muscle tone upon which the
cortically originating voluntary motor command is superimposed.
Each cortical column in the motor cortex is thought to have a facilitatory or inhibitory effect. Activity in horizontal
association fibers from one cortical column to adjacent columns
may provide the integration necessary for appropriate
coordination of agonists and antagonists.
|
 |
 |
Our brain is a mystery and to understand it, you
need to be a neurosurgeon, neuroanatomist and neurophysiologist.
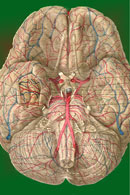
neurosurgery.tv

Please visit this site, where daily neurosurgical activities are going
on.
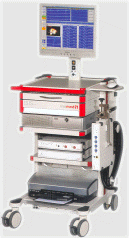
Inomed ISIS IOM System
|
|
|
|